If consciousness were an emergent property of the brain, adding neurons would make you more conscious, and removing neurons would make you less conscious. While we have looked at examples of impaired consciousness, they were related not to the extent but to the location of the damage. This points to consciousness being the product of a system within the brain rather than simply an emergent property from the sheer number of neurons in the brain. If this assumption is true, we can formulate a mechanistic theory of consciousness and (at least on paper) build a conscious system. In this section, we will examine what happens to the sense data after processing in the occipital, parietal, and temporal lobes. Then, we will put the process of consciousness into a diagram.
Mechanistic theory A mechanistic theory is a theory that explains a system as if it were a computer program or a mechanical machine with gearwheels: you can understand it by tracing the input to the output. Pedagogically, a mechanistic theory is of great value as we can explain the essential workings of a system step by step using a diagram with boxes and arrows.
Let us reexamine the idea of the Cartesian theater Instead of arranging the homunculi in an infinite series, we put a finite number of observers into a loop observing each other. Just as you can create a (theoretically near-infinite) number of mirror images by placing two mirrors nearly opposite of each other, we could be somehow fooled by a very high number of loops of consciousness. The mirror images do not exist; they are part of the process of reflecting light back and forth between mirrors. Similarly, we should look at consciousness as a looping system of information (instead of a fixed entity) being perceived, processed, expressed, and perceived again.
Did you know? This process of going back and forth is actually how we form knowledge. By reflecting upon our perceptions, integrating them, and then using that newly found knowledge to reflect upon our own cognition, we get a better and better idea of how the world works. In other words, whenever we learn something new about the world (“what is?”—ontology), we have to reconsider how we learn facts about the world (“how do we know?”—epistemology), and then, with our new way of interpreting what we perceive, again reconsider what we know about the world, and so on. Read more in Philosophy for Heroes: Knowledge.
One candidate for such a loop can be found in the thalamus. The thalamus is not just a relay station with some pre-processing going on. For example, the visual cortex uses the LGN to focus on particular aspects of an image and enhance it. While the LGN sits on the connection between the retinas and the visual cortex, it actually receives 95% of its input from sources other than the eyes, that is:
- The superior colliculus, which is responsible for visual tracking and eye movements;
- The visual cortex which creates a feedback loop with the LGN; and
- The midbrain and brainstem which are responsible for pupillary light reflexes, smooth pursuit of moving objects, the accommodation reflex, antinociception, REM sleep, arousal and sleep-wake cycle, attention and memory, behavioral flexibility, behavioral inhibition and psychological stress, cognitive control, emotions, neuroplasticity, posture, balance, and preparation for fight and flight.
This shows that the LGN pre-processes everything coming from the eyes and is in a feedback loop with the visual cortex. Sense data does not just flow in one direction—bottom-up from the eyes to the visual cortex—there is also information that was already processed in the visual cortex flowing back into the LGN. In both cases, the neocortex provides high-level information to adjust the filters in the eyes and nose. This is similar to the olfactory system. There, the neocortex also “primes” the olfactory system’s cells to increase its sensitivity to particular smells.
While those two examples seem promising, the problem is that each loop degrades the sense data. Like the telephone game where people sit in a circle and whisper the word they (think) they heard from their neighbor, the looping image (or smell) information will no longer resemble the original image captured in our retina (or the original smell captured in our nose).
Still, even without degradation of the signal, we are left with the questions where exactly something like consciousness would arise in this loop, and why we claim to experience it. It is magical thinking to assume that just because something is (potentially) infinite, looping very often, very small, or very large, new properties arise that have nothing to do with the process. For example, when crystals are cooled down, slower, more complicated (but not more complex) structures emerge (see the table with the snow crystals in Philosophy for Heroes: Continuum ). It is just the same rules of geometry and molecular forces applied more often. Similarly, a lot of water droplets are moving when it is raining (complex), but the underlying concept of water falling from the sky is not difficult to understand (not complicated). In summary, just describing a “loop of consciousness” is not enough, we need a precise definition of consciousness. After all, a loop is just that, a loop. Where would the consciousness that we experience come from?
The Process of Consciousness
To map consciousness into a diagram, we start with Figure 6.13. At its core, there are two loops. The first loop simply goes through perception, consciousness, effects on our environment, and back to perception. We observe our own actions (and their effect on our environment) and use this information to think about our next action. Instead of reacting immediately to a new perception, we might first loop “within” our consciousness. This could be the activation of memories, or the mental planning of our next steps.

Now, our job is to refine this diagram until we have replaced all occurrences of “consciousness” with actual brain parts. For the perception part, we have cleared up how information arrives in the visual cortex through the eyes and is then processed in the parietal lobe (identifying the “where”) and temporal lobe (identifying the “what”). This process (see Figure 6.14) is as follows:
- The apple reflects light rays which hit the retina of our eyes.
- The optic chiasm combines the nerve signals from the retina into the left and right visual fields.
- The thalamus pre-processes and then recombines the image data with possibly other sensory data.
- The visual cortex receives the visual information.
- The parietal cortex (“where”) and temporal cortex (“what”) analyzes the data.

Working Memory
How do we access the brain’s sense data?
Memory addresses the issue that sense data processed by the brain is fleeting. A basic approach nature took to remember previously perceived sense data was to release stress hormones, preparing our body for possible injury, and also to take one of the four actions: fight (proceed), flight (run away), freeze (try to not be seen), or fawn (try to please—more applicable in confrontation with other members of the same species). The chemicals will last for a longer time in the body, long enough to deal with the situation at hand—a kind of short-term memory.
With the analogy of neural committees, memory can be thought as every member of the committees being put into one of those four emotional states. The committees will still not remember, for example, a tiger, but they continue to stay in a fearful state until the hormones have subsided. Besides its function as memory, the hormonal system can also act like a prefrontal cortex and suppress other signals. For example, when running from a tiger, it would be good to suppress pain and thus you can keep going despite an injured leg.
In computers, so-called “regenerative capacitor memory” were used as a memory system. It was based on electrical properties of capacitors which you can “load” with a single bit (load or no load) and which keep that information for a while. To prevent the degradation of the memory caused by leaking of energy over time, the capacitor is regularly refreshed with its own bit-value. In the brain, we find a similar mechanism: to keep a thought pattern active, the solution is to continuously “read” and then immediately “write” the pattern back again, forming a loop and providing memory that can be accessed at any time. You can even create such a loop yourself to extend your short-term memory: simply tell yourself aloud the thing you want to remember again and again to keep it as an active thought pattern in your brain. The downside of this kind of memory is that, just like with the previously mentioned telephone game, the signal degrades with each loop.
In the brain, we have a variety of distinct memory systems based on loops that refresh themselves and thus allow retaining information for a longer amount of time:
Phonological loop The phonological loop is an acoustic memory system of the brain that holds spoken words or sounds. It involves (among other brain parts, see Buchsbaum and D’Esposito [2008]) Broca’s area and Wernicke’s area.
Viso-spatial scratchpad The visuo-spatial scratchpad is responsible for temporarily storing visual and spatial information (involving the occipital lobes).
Episodic buffer The episodic buffer is responsible for remembering the sequence of events, including the last state of an entity.
That these types of memory are separate can easily be demonstrated by trying to remember a phone number. By imagining one part of the phone number visually (using your visuo-spatial scratchpad), and the other part of the phone number as individual numbers repeating them with your inner voice (using the phonological loop), you can remember phone numbers more easily, at least in the short-term. Together, all those types of (short-term) memory form the so-called “working memory” [Chai et al., 2018]. This is also the reason older phone numbers contained letters for better memorization, using the printed letters below each number on the telephone.
Working memory Working memory is the collection of a number of different (limited) short-term memory systems in the brain. The prefrontal cortex has access to the working memory.
Adding working memory to our diagram results in Figure 6.15:
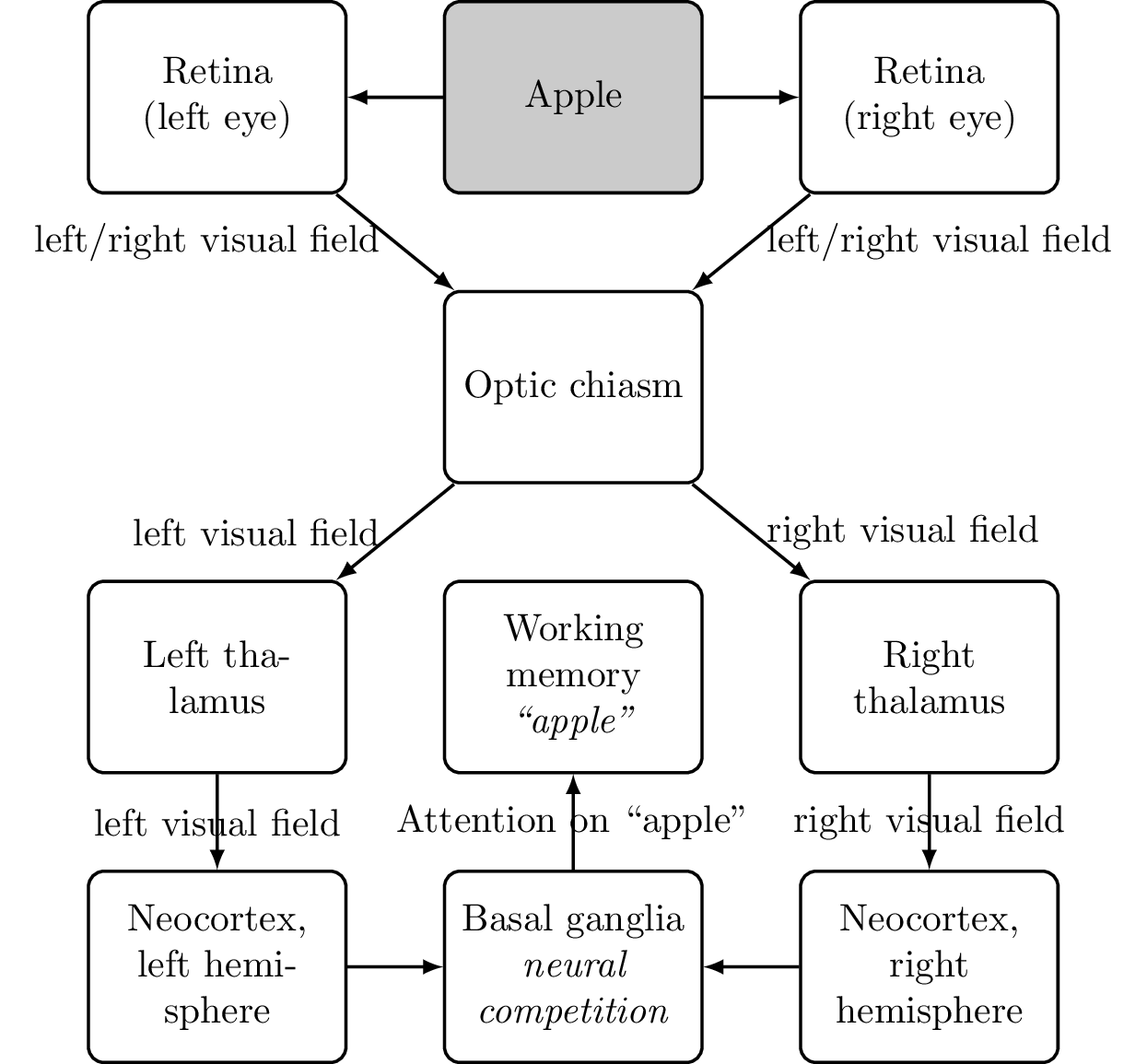
We also have what is called declarative memory which connects long-term memories of sense data. We can find it in the amygdala (mapping thalamic sense input to emotions), and the hippocampus (connecting locations with each other for orientation and pathfinding). The latter has been shown by experiments with rats where specific neurons in the hippocampus became active when the rat was in certain locations [Skaggs and McNaughton, 1998]. The hippocampus is also involved in creating long-term memories in the neocortex. An example would be learning associations, that is, to connect two concepts with each other.
Declarative memory Declarative memory connects one memory with another. For example, the amygdala maps thalamic sense input to emotions; the hippocampus maps places with each other for orientation; and the neocortex maps concepts with other concepts (a tree is a plant).
Declarative memory is processed by and temporarily stored in the hippocampus. Mainly during sleep, it is then copied for long-term storage into the temporal lobe and other places. Someone with damage to his hippocampus has a greatly reduced ability to form new declarative long-term memories. The affected person tends to forget what he did 15 minutes ago, and may repeat what he did just before [Squire, 2009]. But he can still use his working memory as usual and form new non-declarative memories. For example, he can learn new motor programs in the basal ganglia and cerebellum, or learn to recognize new images.
As concepts are knowledge about the world and seldom subject to change, they are stored differently than working memory. Instead of continuously looping thought patterns through the brain, neurons are trained repeatedly to adapt their configuration during REM sleep (dreaming). This can be imagined like a stream of water eventually digging a river bed into the ground: the more often a particular memory is replayed, the stronger the impact on the involved neurons. If a particular memory is connected to a strong emotional experience, this effect is magnified even more. Similarly, implicit memories like muscle memory are encoded in the configuration of individual neurons (in the basal ganglia and cerebellum), too, and the strength of the memories increases with repetition of the motor program.
While declarative memory is passive, external stimuli can activate a cascade where activating one memory leads to the next and so on. This applies especially to odors because of the direct connection of our olfactory system to the neocortex (and thus to memories). Consciously recalling memories works the same way: instead of recalling memories directly, we have to work through our memories step by step and find things we associate with a memory we want to recall. For this, the prefrontal cortex sends signals to the hippocampus to simulate sense data that would normally lead us to remember a particular thing [Minxha et al., 2020]. The hippocampus then activates the neurons related to sense data in the temporal cortex. In that regard, the same pathway is used whether you see something that leads you to remember something (going from the thalamus to the hippocampus to the temporal cortex) or imagine something (simulating the sense data with the help of the prefrontal cortex, going from the prefrontal cortex to the hippocampus). You could also use imagination to activate your visual cortex, working memory, thalamus, and ultimately your hippocampus. For example, when you are wondering where you have left your keys, you cannot ask your memory to magically provide you with the location (assuming you do not have a special place where you always place your keys). You have to trace your steps and ask yourself where, for example, the keys were when you were in the kitchen. By imagining the kitchen table, maybe you remember the jacket you have left in the kitchen, and that a jacket pocket contains the keys.
"One takeaway is that memories are not movies we can simply replay but always scenarios we have to reconstruct piece by piece. In fact, we use the same brain parts to imagine a future scenario as we are using to recall a past memory." [Schacter et al., 2012].
Stream of Consciousness
What happens to the sense data after it is processed in the occipital lobe, parietal lobe, and temporal lobe?
The TPJ combines the ventral (“what”) and dorsal (“where”) streams to construct information about the subject (“Who is perceiving this?” “Whose thoughts are these?”). This data is then grouped together with the rest of the information from the parietal and temporal lobes into the working memory for high-level processing. For example, let us imagine a scenario of two people (Anna and Peter) in a room with an apple on a table. Peter is looking at the apple, and Anna is looking at Peter and the apple. Also, Anna is wearing a hat and is in love with Peter. Then the contents of Anna’s working memory would be as listed in Figure 6.16.
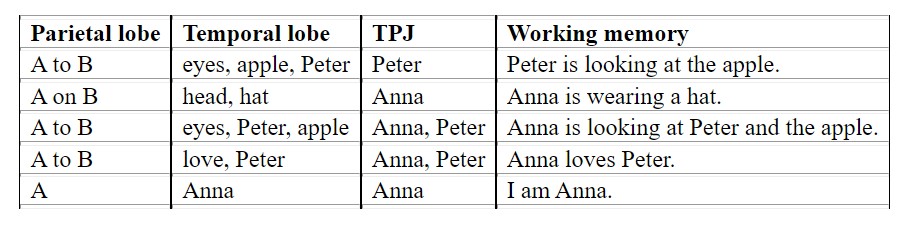
Whether you ask another person, or another person asks you, in both cases, your brain hears the question “What do you see?” and your brain has to determine who is asking whom. Similarly, when answering the question, there might be multiple entries in your working memory about what is seen by whom. There is not only a list of items you see, but also a list of items the other person might see. When looking into someone’s eyes and that person is looking into yours, your working memory has (at least) two entries: “I see you” and “You see me.” Hence, each sentence contains not only raw sense data, but also information about who is perceiving the sense data.
The point is that by looking at Peter, Anna knows that he is, for example, looking at an apple. She has no direct access to his sense data but infers this information from the direction of his gaze. While the subjective experience between this abstract inferred information (“Peter is looking at an apple”) and her own sense data (looking at Peter and the apple) is fundamentally different, this does not matter on the level of abstraction of the working memory. There, it is reduced to simply “Peter is looking at an apple” and “Anna is looking at Peter and an apple.”
Without this additional information, the prefrontal cortex would not know what the real-world equivalent of the word “Anna” is. Likewise, without processing by the TPJ, Anna would not know who is looking at or loving whom. She would be unaware that Peter is looking at the apple, that she herself is wearing a hat, that she is looking at Peter and the apple, or even that she loves Peter. She might still act positively toward Peter, but she could not use that information for any higher processing like language, planning for the next day, or thinking about the social appropriateness of her actions. If the information is not completely missing but miscalculated or misattributed by her TPJ, she might end up (maybe falsely) thinking that her own emotions (also) belong to Peter (“Peter loves Anna”).
If the brain does not correctly attribute sense data as being perceived by the self, it is not put into one’s working memory (or it is attributed to someone else). This situation is similar to when something does not catch our interest in the temporal or parietal lobe: the corresponding sense data is filtered out. For example, in the “Where’s Waldo?” children’s puzzle book series, the reader is challenged to find a character named Waldo hidden in a group of dozens of people. The entire picture is available to the eyes but most of the details are filtered out by the brain. Only by scanning one part of the image after the next, Waldo can be found.
It could also lead to false memories: someone telling us about an event might turn into what feels like a first-hand experience in our memory. Likewise, this could be the origin of ghost stories or out-of-body experiences: attributing a voice or appearance to something outside the body instead of to oneself can cause serious emotional distress. The fact that we need an attribution of self to sense data also explains how short-term memory impairments can stem from TPJ damage rather than damage to parts of the brain that actually store working memory [Ravizza et al., 2011].
This idea of a third stream besides the ventral and dorsal stream also helps us to better understand how AHS can develop from damage to the rTPJ (or to connections from or to the rTPJ). The brain still receives visual and sense information from the left arm, but that information is never processed in the rTPJ for integration. The working memory will contain information that there is an arm, and that it is moving, but not information about whose arm it is. Eventually, the brain might conclude that it is not your arm. Any action initiated by the parietal lobe (utilization behavior) will then no longer be checked by the prefrontal cortex. The arm might end up acting out socially unacceptable or even self-harming behavior.
While the rTPJ is needed to recognize the difference between self and others and to properly attribute sense data to entities, the lTPJ translates this information into something that can be used by other parts of the brain. Remembering what we have discussed about the difference between the left and right hemispheres, the left side is responsible for abstract language while the right side is about spatial relationships. To be able to speak about an object you are seeing and to use this abstract information to plan ahead, the lTPJ needs to be involved.
Another way to understand this is to look at the lTPJ as being responsible for concepts, while the rTPJ is responsible for measurements. You might be aware (with the lTPJ) that Peter, an apple, and the process of looking are involved. But you need the rTPJ to understand that Peter is another person, which person Peter in your field of view is, where the apple lies in relation to Peter, and that Peter is looking at the apple.

The Executive Functions
Having explained how sense data arrives in the working memory on the one side, we can narrow down the definition of consciousness by examining the other side, namely the last steps leading up to a physical action. If it is not a reflex or other non-conscious action (breathing, swallowing, coughing, etc.), the primary motor cortex generates motor programs to achieve a goal. The basal ganglia select a single motor program and coordinate the final movement program with the cerebellum. The selection process relates to the basic theory of how we make decisions that we have explained in Philosophy for Heroes: Continuum : competing neural committees refine the motor program in several steps and the “best” program is selected. If the motor program is new, this process can take a moment (or could take multiple physical attempts if the movement is complex, like a child trying to walk for the first time).
The executive functions are provided by the prefrontal cortex. Instead of initiating an action, the main task of the prefrontal cortex core function is to suppress whatever catches your attention if that action does not align with your long-term plans or personal preferences. For example, not eating the candy bar in front of you to fulfill your long-term goal of being healthy requires prefrontal cortex activity. Additionally, instead of suppressing a single action, the prefrontal cortex can also suppress every activity but one. This can help with focusing on one particular activity. But instead of trying to directly suppress most neural activity in the primary motor cortex, the actual suppression happens in concert with the basal ganglia. Damage to the basal ganglia is one of many causes that can lead to a loss of motivation (apathy): while the primary motor cortex still generates several programs, there is no arbiter to select the best one. Even with focus of the prefrontal cortex, this might not be sufficient to create a strong enough signal to execute an actual physical action.
Executive functions The executive functions represent a series of means by which the prefrontal cortex can suppress thought patterns in other parts of the brain. While winners in the neural competition are selected with the help of the basal ganglia, the prefrontal cortex can counteract those decisions in favor of other actions. The prefrontal cortex makes these decisions based on its models. For example, stealing goes against the norms of society, so the prefrontal cortex suppresses the (utilization behavior of the parietal lobe’s) urge to grab someone else’s property.
As a compromise between focus, energy usage, and the possibility to quickly react to your environment, the brain actually oscillates between the parietal lobe (utilization behavior) and frontal lobe (focus) activity [Misselhorn et al., 2019]. This way, while the prefrontal cortex focuses on the current action, the neurons in the parietal lobe are not firing and vice versa. A healthy prefrontal cortex would suppress distractions, or interrupt activities that go on for too long (in favor of being able to pursue multiple goals and react to the environment). Impairments to the prefrontal cortex and its communication with the rest of the brain can manifest as Attention Deficit Disorder (ADD or ADHD). It leads both to a lack of focus and to jumping between different tasks, as well as to “hyperfocus” when the afflicted person focuses on one activity for a long period of time.
We have learned that with a damaged prefrontal cortex, we would tend to show utilization behavior, which means applying action to anything in our environment that catches our attention (e.g., eating the candy bar). What initiates utilization behavior is the parietal lobe, based on what has been provided by the senses and processed in the occipital and temporal lobes. This is how most animals (and human children) react toward their environments if their prefrontal cortex is not as developed as it is in (adult) humans. When an animal is presented food, the animal eats it. When the animal is shown a toy, it plays with it. When we present a smartphone to someone with a short attention span, he might feel compelled to pick it up and check his messages, even if doing so is inappropriate in a given setting.
One can also imagine the executive function like a separate group in our neural committees. This new group takes care that, once started, activities are not stopped but brought to a finish. For example, eating the candy bar might be the most interesting thing, but finishing a work assignment might save us some time: interrupting work is often connected with a lot of overhead because you have to start thinking about it again from the beginning. Instead of going back and forth, our brain focuses on one thing, finishes it, then moves on to the next.
After an action has been executed, the thought pattern originally causing the action will be suppressed. For example, when standing in front of a sink and seeing a piece of soap, we might be inclined to wash our hands (utilization behavior). If our prefrontal cortex does not intervene, we will wash our hands. Once finished, our prefrontal cortex will then send a signal to suppress washing our hands a second time—it has evaluated our hands as now being “clean” and “safe.” If this signal fails (which it sometimes does, for example in sufferers of OCD), the brain’s internal status of the hands still shows that they are dirty, so we wash them again. Sufferers of phantom limb pain face a similar problem. Without the signal that the limb has been unclenched, the internal status remains unchanged. For both sufferers of OCD and phantom limb pain, one could argue that they could just look at their hands or missing limb. As we have discovered previosuly, the brain requires two sources of information to update its body schema. In the case of phantom limb pain, it is the visual signal (with the help of a mirror) and the initiation of muscle movement (unclenching). In the case of OCD, it is the reevaluation of the risk together with the act of handwashing.
We can test this suppressive effect of the executive function by measuring the delay in reaction time between congruent and incongruent stimuli. For example, the word “blue” printed in red font has a different meaning than its color (incongruent stimulus). It is more difficult to process than the word “blue” printed in blue font (congruent stimulus). To experience it yourself, take a look at Figure 6.18 and try to name the color of each word instead of reading the word. To give the correct answer, the prefrontal cortex has to suppress the part of the brain that provides the meaning of a word (“blue”) in favor of the part of the brain that provides the information about the color of the text (which may be, for example, red).

The Inner Voice and Mind’s Eye
Depending on the strength of the connections in the brain, the ability to imagine things can differ from person to person. For example, people with aphantasia do not have a “mind’s eye.” They lack visual imagination. They might still have spatial imagination to figure out where in the house to put a flower vase, but they cannot consciously imagine whether it would fit in terms of colors or form. They might have an abstract knowledge about what types of plants, colors, and vases they have in their home, but they cannot conjure a combined image and describe it.
This is similar to how we cannot imagine smells because the sense of smell is not connected to the system in the brain that creates imaginations. This is an indication that the sense of smell is wired differently when it comes to this inner experience. This is supported by the fact that the sense of smell remains active even when a person is otherwise unconscious in a coma because of a damaged thalamus [Arzi et al., 2020]. We can also make memories of smells without consciously being aware of them. Both facts point to the olfactory system being able to bypass the thalamus and send signals directly into the neocortex.
For people with hyperphantasia, even textual descriptions can evoke a rich inner visual imagery, sometimes referred to as “mental cinema.” fMRI studies suggest that hyperphantasia is related to a high activity in the visual cortex and low activity in the prefrontal cortex, while aphantasia is related to a high activity in the prefrontal cortex and a low activity in the visual cortex [Fulford et al., 2018]. Similarly, it seems that a strong mental focus on the mind’s eye or inner voice can suppress rTPJ activity and induce inattentional blindness (similar to hemispatial neglect or blindsight but not caused by damage to the brain) or deafness [Todd et al., 2005]. This is in line with our own experience where daydreaming can lead us to no longer pay attention, despite our eyes being open. This might be the reason people tend to look into the sky when thinking about something and using their imagination. This frees up processing power in the visual cortex as signals no longer have to compete with direct sense data.
People with hyperphantasia are aware that what they are experiencing are imaginations and not hallucinations. This is because their brain has the information that a particular imagination was initiated by themselves. This is different from people suffering from a tumour near their thalamus. It can generate nerve impulses that cause the experience of, for example, hearing voices. Without the information that the words were either created in Wernicke’s area or spoken by another person, the brain can end up assuming that the voices must come from a supernatural source [Dutschke et al., 2017].
In summary, it seems that the thalamus, visual cortex, and prefrontal cortex are involved in imagination. So, the surprisingly simple solution to explain the inner voice or mind’s eye is that it is a description of anything transferred to the thalamus. Given that we are not constantly flooded with pictures or sounds (some people are—for example, the filtering mechanism for people on the autism spectrum is limited), this forwarding is regulated. A thought “pops up in our mind” if it hits a certain threshold—and this is arbitrated by the basal ganglia. In that regard, the “stream of consciousness” is a list of the most dominant thoughts at a given time. While this still does not answer how the thalamus can experience anything, we can update our diagram of consciousness (Figure 6.19):
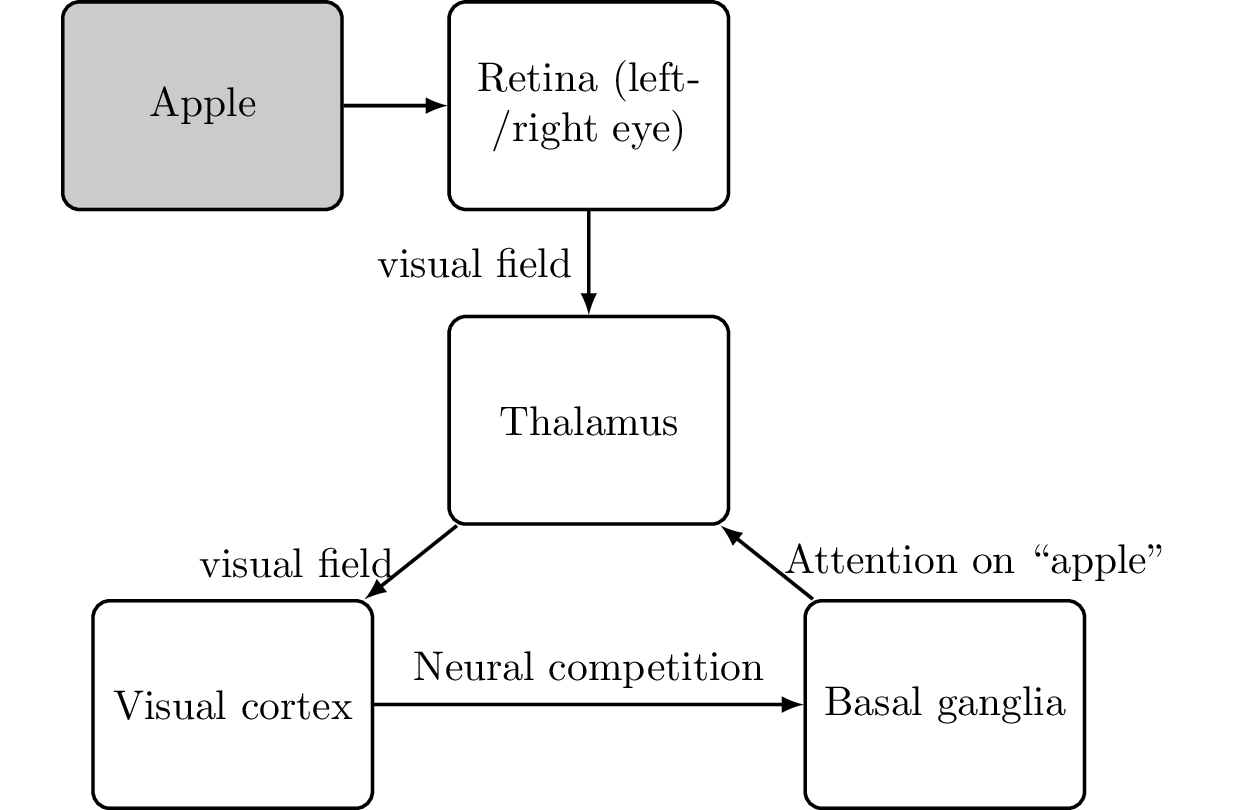
Logically, if we somehow shared our thalamus with another person, they could listen in to what we call our inner voice. We know at least of one case of conjoined twins (Krista and Tatiana Hogen) who share the thalamus but have separate neocortexes (see Figure 6.20).
.png)
Besides being able to perceive what the other twin perceives with her sense of sight, auditory sense, or sense of touch, each twin can perceive what the other twin is thinking [Squair and Squair, 2012]. For example, what one twin sees is still processed in her own visual cortex. But as the sense data passes through the thalamus, it is also processed in the visual cortex of the other twin. Just like people with a damaged corpus callosum, the twins do not share a direct connection between their neocortices. In that regard, they only perceive the results of the thought processes of their twin—just as if they said out loud whatever came into their minds (thalamus). So, just because their loop of consciousness is connected, this does not mean that they actually think together on one problem like our two hemispheres do with the help of the corpus callosum. In a way, both hemispheres are like two singers. As long as they hear each other, they can easily synchronize with each other. If they are disconnected, they lose the beat and can only coordinate with each other what song they will sing next. What happens during a “song” (loop of consciousness) is not synchronized.
Now, from the thalamus, the information is wired back into the cortex depending on the type of information (for example, imagining an apple activates the visual cortex). There, it again can take part in the neural competition and might again emerge as a winner. This forms the so-called cortico-basal ganglia-thalamo-cortical loop or, as we will refer to it in short, the loop of consciousness.
Loop of consciousness The loop of consciousness refers to a network of connections in the brain from the thalamus to the cortex, then to the basal ganglia, and then back to the thalamus (also called the cortico-basal ganglia-thalamo-cortical loop). It is believed that this is the source of our subjective experience of the world.
This loop-like structure also explains why our inner experience of consciousness is linear: before we can think about a thought we just had, it has to be routed through the basal ganglia and thalamus to arrive back in the cortex. This also applies to split-brain patients whose left and right hemispheres are not connected by the corpus callosum. While the left and right side of the thalamus are conjoined, there are no direct nerve connections between the left and right hemispheres of the neocortex. In that regard, the split-brain patient’s consciousnesses are linear, too, but they have two separate loops. Someone with a split-brain syndrome is very similar to the conjoined twins Krista and Tatiana Hogen: there is no synchronization between the hemispheres, but the loop of consciousness of both hemispheres pass through the same thalamus (see Figure 6.21). The main difference is that each hemisphere of a split brain is incomplete, while in the case of the conjoined twins, they each have a left and right hemisphere.
.png)
Having now gained an understanding of the role of the two hemispheres, the corpus callosum, the thalamus in creating the “inner voice” and “mind’s eye,” and how the working memory is filled, the next step is to examine more closely how the prefrontal cortex processes the information in the working memory.