This is an excerpt from the book series Philosophy for Heroes: Continuum.
Many Worlds Interpretation
Why could the many worlds interpretation be called ontologically wasteful?
An approach to explain what is going on at the ontological level is the so-called many worlds interpretation. Instead of claiming that until something is measured, it is not defined, it states that at all times, we have a definite history. This means that Schrödinger’s cat is always either alive or dead, no matter whether you open the box or leave it closed. In that regard, the many worlds interpretation is deterministic. There is a clear path that leads from a past situation A to the current situation B.
But in order to be deterministic, it needs to do away with another property of classical mechanics, namely that there is but one reality. One needs to assume that with every measurement (interaction between particles), the universe splits into an infinite number of copies, each copy representing one possible outcome of the probability of the measurement. Looking toward the past, you always have but one “parent universe.” Looking toward the future, you always have an infinite number of universes. In the case of Schrödinger’s cat, you can imagine that the cat stays alive in at least one branch of this tree of possibilities. While it becomes deterministic by using this “trick,” you still do not learn anything about how the universe actually works (there are no cause-action pairs). Ontologically, the universe would be still based on probabilities—you do not know why your universe “decided” to pick a certain branch.
When talking about universes, we have talked about the infinite universe, as well as the idea of having an infinite number of universes. The universe is a large (and possibly infinite) canvas, with big bangs at random places. If we could look at this canvas from afar from outside the big bangs, we would see independent “pocket universes” here and there, like stars in the night sky. You could theoretically travel from one pocket universe to the other if you traveled long enough. But the many worlds interpretation refers to a different type of “infinite” or “many.” The branching in themany worlds interpretation does not refer to those pocket universes, it means whole worlds, which in principle (and not because they are so far away) could not interact with each other. So, to summarize:
- Universe: the infinite canvas on which big bangs (creating pocket universes) happen.
- Pocket universe: created by big bangs and refers to the observable universe.
- Many worlds: distinct universes which, in principle, cannot interact with each other. They are created with every measurement.
While this approach sounds far-fetched, it is one of the many possible interpretations of the observations from experiments related to quantum theory. It relies on the same proven mathematical results we get when using other interpretations. What is obviously different is its ontology. It does assign a definite state to all entities at every point in time. But it is an extremely wasteful interpretation, violating the Occam’s Razor principle to the largest extent: with each interaction on the quantum level, it creates an infinite number of copies of the universe. And with infinity, strangeness ensues: with all the possibilities becoming a reality in some universe, you will have one universe with a basically immortal cat, defying all odds. In contrast, with the Copenhagen interpretation, the survival rate of the cat would tend toward zero.
Instead of saying that the quantum world is based on probabilities, in the many worlds interpretation, it is argued that the universe is fully deterministic—insofar as it has a definite history—but that at each “moment,” the universe splits into an infinite number of copies, each representing one choice among the probabilities. It would be ontologically wasteful as it requires the creation of an infinite number of universes. In addition, you would still not know why a certain branch was chosen.
Hidden Variables
What sets the hidden variables interpretation apart from the Copenhagen interpretation of quantum mechanics?
In the Copenhagen interpretation, the state of the universe is “undefined” before it is measured. That is what “quantum weirdness” is largely about, precisely because that is not how probabilities usually work. “Schrödinger’s cat” was all about highlighting the weirdness of the Copenhagen interpretation, by taking the supposedly undefined state at the quantum level to the macroscopic level: a cat that would be both dead and alive.
So, a different approach does away with the whole idea of probabilities. Instead of assuming that the collapse of the wave function is based on probabilities or chance (or branches off into infinite worlds), it assumes that there are “hidden variables.” According to this idea, the process is not truly random, but we simply lack an insight into the hidden workings of the system we are measuring. For example, there is a 1/6 chance to roll a 7 with a pair of dice, but there is a 100% “chance” for one result to show up when it follows from the previous state. If you knew the exact position of the dice as well as the vector of the dice throw, you could calculate the result.
Unfortunately, when using hidden variables to do away with the probabilities, we need to introduce a different kind of “weirdness”: non-locality. Non-locality is the idea that an action at one place influences something at another immediately. But at its core, non-locality is a simple concept and not “weird” at all.
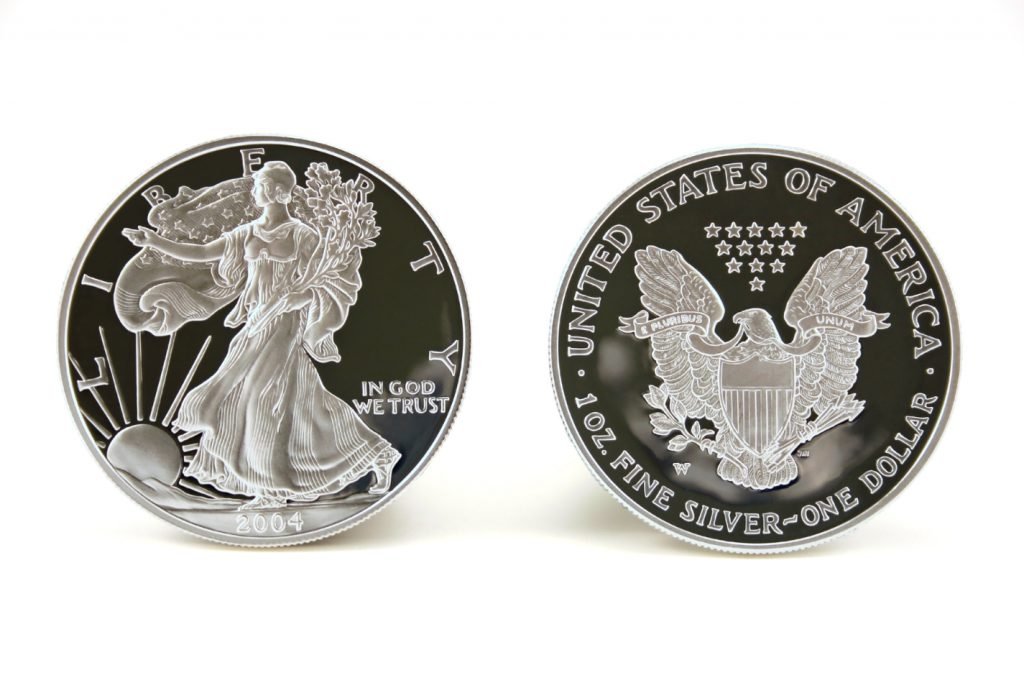
Suppose I, without looking, take a coin and split it horizontally in two. Then, still with neither one of us looking, I give you one half and leave on a journey to the stars. The first person who looks at the coin immediately knows which part the other person has, despite being light-years away. There has been no “faster than light” communication, yet our knowledge about the other half of the coin was instantaneous over light-years.
Non-locality seems surprising only if you approach nature with the worldview of the Copenhagen interpretation, namely that nature waits until you take a look at her. If you assume that the coin halves had no definite property until they are measured, and that the fundamental properties of the world supposedly consist of mathematical probabilities that are only realized when being measured, you might find this “instant” communication between the coin halves as some sort of “spooky action at a distance” that seemingly violates the limits of the speed of light.
The Bohm interpretation sees the quantum world instead as particles guided by a “pilot wave.” This wave has properties and constitutes what we previously have called the “hidden variable.” Just like the halved coin, a wave is intrinsically non-local: you throw a stone into the water and the corresponding wave contains the information of the stone throw. No matter how far it travels, the information about the initial throw is carried to multiple places. In the case of the quantum level, it is “hidden”—maybe hard or impossible to measure, but it is (ontologically) there. The place where the stone hits the water could be compared to the moment where both coin-carriers part ways, and the “wave” is the person traveling away with half of the coin. Looking at one half of the coin tells you of course instantly something about the other half of the coin because both halves are part of the same wave (“entangled”) which was created by a common “throw” (the division of the coin).
Scientists have found it difficult to understand the Copenhagen interpretation as it does not explain where the probabilities come from. The hidden variables interpretation replaces the probabilities with a fixed “hidden” cause, the so-called pilot wave. It might be hard or impossible to measure, but they are (ontologically) there. As such, the hidden variables interpretation is deterministic—as opposed to the Copenhagen interpretation.
Quantum Effects on a Macroscopic Scale

With the help of a vibrating bath of silicone oil and individual droplets, quantum effects could be simulated on a macroscopic scale (see Figure 3.16). The vibration of the bath allows droplets to hover over a packet of air just above the surface for a long time. Below the droplet, there are waves. A moving droplet will ride along its waves, moving in directions or seemingly randomly. Surprisingly, tracing these paths, a pattern emerges that is very similar to that of particles at the quantum level. When these droplets hit a double-slit experiment, they do not act like the previously mentioned billiard balls. Instead, they act like a wave (see Figure 3.17). The guiding wave they take with them while floating over the water bath influences their path through the slits, just like it was observed with particles at the quantum scale.

Outlook
Due to what we have said about Heisenberg’s uncertainty principle, we cannot tell if a particle either does not have both impulse and a location and simply acts according to probabilities, or if there is some kind of “hidden variable” within a particle. But what is the “mechanism” within a particle? If we cannot peek into the particle and its workings, how can we tell if there is some random factor involved?
The major challenge in science is that when looking at the universe, we are always part of what we are looking at. We cannot put the universe under a microscope and look at the universe from the outside. It is us, the universe, that looks at itself. In physics, this problem has led to two approaches: description of the probabilities of how particles behave on the one hand, and, on the other, interpretation of the underlying reasons that these probabilities appear.
The first approach simply focuses on the math of everything related to this “jiggering” on the particle level. From that, the so-called “quantum theory” was born. It is one of the most tested theories in science and has never been proven wrong in the 100 years since its development. With it, you can compute the pattern that a stream of particles will take. This approach does not “peek into the box” but simply assumes that at a certain level, the world is ruled by probabilities, with no internal or external state influencing them.
The second approach deals with the interpretations of the quantum theory. Scientists want to know how it actually works, they want to understand it. In physics, this has led to a number of interpretations of the universe. In a way, it is another example for the list of theories we have discussed in the context of Occam’s Razor: there are an infinite number of interpretations of a phenomena, but we want the one that does not add unnecessary baggage.
Example We can look at the universe with the Earth as the center and all the planets and stars moving around it in strange paths. Or we simplify the model and have the Earth and planets orbiting the sun in nice ellipses. We can look at the Earth as a planet with a (more or less) planar space-time, or we look at the Earth as a hollow sphere with us living on the inside and with space-time stretched in a way that the whole universe is condensed in the center of the sphere.
All interpretations certainly require an adaption of our basic philosophy. But just because quantum mechanics is based on mathematics to make predictions, and just because many of today’s technologies are based on the results of quantum mechanics, we do not have to accept all the weirdness that comes with the Copenhagen interpretation. It is certainly determinism that is most compatible with the philosophy outlined in the first book of the series, Philosophy for Heroes: Knowledge, and thus also the foundations of science.
The unanswered question for non-deterministic interpretations is how they can arrive at their conclusions while not at the same time undermining the philosophic requirements of the very scientific method they are using. Taken to their conclusion, for example the Copenhagen interpretation has to introduce either an arbitrary distinction between the micro and macro world, or an ultimate observer—a “God”—that observes the observers in order for them to exist. You end up with the same mathematical results, but again introduce a lot more ontological baggage as opposed to deterministic interpretations like we have laid out here.
Either way, I hope that the discussion here has shown one central point: that we are dealing always with interpretations, not (testable) theories. They are philosophical explanations to explain laws of physics. At this point, no interpretation really has the upper hand and we should wait until more research has been done. At least the simple two-dimensional model from above seems to be a good candidate that could serve as a model to understand what is happening at the microscopic level. With this model and the deterministic Bohm interpretation, it looks like science is getting closer to a reasonable model for quantum mechanics.
With a hope for a rational explanation in mind, we should not shy away from topics like these. All topics can be learned and understood given enough time and nobody should get the upper hand in a discussion just because he or she uses big but undefined words—like pointing to the dragons on the map, as if they would give them power to make proclamations about the world without having to base them on testable facts.
Summary
Instead of saying that the quantum world is based on probabilities, in the many worlds interpretation, it is argued that the universe is fully deterministic—insofar as it has a definite history—but that at each “moment,” the universe splits into an infinite number of copies, each representing one choice among the probabilities. It would be ontologically wasteful as it requires the creation of an infinite number of universes. In addition, you would still not know why a certain branch was chosen.
Scientists have found it difficult to understand the Copenhagen interpretation as it does not explain where the probabilities come from. The hidden variables interpretation replaces the probabilities with a fixed “hidden” cause, the so-called pilot wave. It might be hard or impossible to measure, but they are (ontologically) there. As such, the hidden variables interpretation is deterministic—as opposed to the Copenhagen interpretation.