This is an excerpt from the book series Philosophy for Heroes: Continuum.
“Well, in our country,” said Alice, still panting a little, “you’d generally get to somewhere else—if you run very fast for a long time, as we’ve been doing.”
“A slow sort of country!” said the Queen. “Now, here, you see, it takes all the running you can do, to keep in the same place. If you want to get somewhere else, you must run at least twice as fast as that!” —Through the Looking-Glass [Carroll, 2015, p. 27]
THE RED QUEEN HYPOTHESIS · “Red Queen” is a reference the novel Through the Looking-Glass where the protagonist needs to run just to keep in the same place. The Red Queen Hypothesis refers to a stable balance between two competing species that are interlocked in their evolution and where both are evolving, yet no side gets the upper hand. They are bound to each other in a way that the failure of one species is the success of the other. A gazelle failing to outrun a cheetah ultimately helps the cheetah to procreate. Likewise, a cheetah failing to catch a gazelle helps the gazelle to procreate. Another example would be plants and caterpillars, with the plants producing more and more toxic leaves, while the caterpillars become more and more resistant to the toxin. Over time, both species become increasingly specialized in catching or evading each other.

Genetic evolution is the tool of choice for nature to adapt to changes in the environment. For larger animals, there is an evolutionary race going on between predators and their prey: the slowest prey is killed by the fastest predators. If a mutation improves speed on either side, it puts evolutionary pressure on the other. If, for whatever reason, one side ever gained a very significant advantage, the hunt in the next generation would be so successful that only a few prey animals would be left. The predators would end up starving due to their own success and the balance would slowly return as only the very best prey survived.
For example, the speed of a gazelle cannot really compare to the speed of a cheetah, but the cheetah can hold its speed only for a very short time. So, the cheetah has to sneak close enough to the gazelle for the hunt to be successful. If the eyes of the gazelle improved, the camouflage of the cheetah would have to improve. Those gazelles that saw the cheetah approaching would survive, while those that did not would end up the victims of the cheetah. In the case of gazelles, the survivors pass on their genes to the next generation, which will be more careful, faster, or more endurant. Meanwhile, the cheetahs that are faster, better camouflaged, or more stealthy pass on their genes to the next generation. Given no significant changes in the environment, this kind of evolutionary race can go on forever. But because the lifespan of a cheetah is similar to that of a gazelle, neither side gets the upper hand; the speed of genetic changes on both sides is very similar, too.
The case of an evolutionary race is quite different when it comes to humans and the viruses that “attack” us. The generation cycle of viruses is only a few hours, compared to humans’ two decades. Thus, a virus can adapt to our genetics 100,000 times faster than our genetic code can adapt to the virus. In addition, there are more than 200 different (known) viruses that can infect humans. With these odds, a more adaptive system than sexual recombination of our DNA is necessary. To understand how it is even possible that animals with such long generation cycles (including humans) could survive in the presence of viruses, we need to take a look at the basic workings of the human immune system. We will look at a simplified model of the immune system.
The Innate Immune System
The so-called “innate immune system” is based on our DNA and provides physical protection against pathogens. Most pathogens can never reach our organs or bloodstream because our skin and mucus (in our nose, mouth, and lungs) physically and chemically block anything from entering the body from the outside. In that regard, the human body evades an evolutionary race with the pathogen on a genetic level: it does not matter what structure the virus has, it cannot pass through barriers such as mucus. Exceptions to the rule would be sexually transmitted diseases or pathogens transmitted by insects (or generally, bites by any type of animal) that bypass mucus or skin.
The second line of defense is inflammation. If the body detects that the outer defense was breached (e.g., because of a wound), the affected area becomes inflamed. So-called “macrophages” (see Figure 4.24) call on neutrophil cells to help destroy incoming pathogens—as well as healthy cells nearby, just to be on the safe side. The neutrophil cells use a variety of strong oxidizing agents like hydrogen peroxide or hypochlorite to chemically destroy the structure of a pathogen.
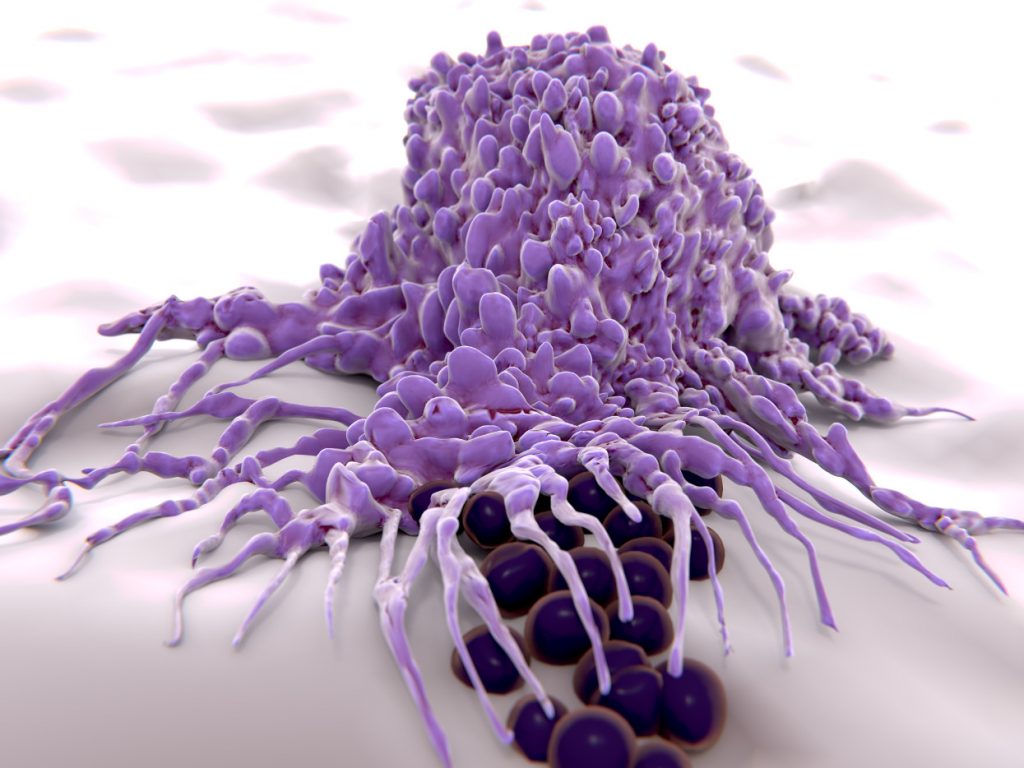
Beyond coordinating inflammation, macrophages have wide-ranging abilities. For our discussion, it is relevant only that they seek out pathogens (as well as dying body cells). The macrophages encounter and engulf the pathogens, break them down chemically, coordinate the neutrophil response, and present parts of the pathogen structure to the adaptive immune system.
The drawback of this system is that neutrophils come to any injured tissue, even internal injuries that do not have a chance of getting infected by external pathogens. This is a design flaw. The culprits are mitochondria which reside in our cells, powering chemical processes in a symbiotic relationship. There, they also hide from the immune system. But when a cell dies, the mitochondria get spilled into the bloodstream. While this sounds harmless, mitochondria being part of animal cells for billions of years, they are still recognized by the body as a foreign entity that has to be killed and that activates an innate immune response, including unnecessary, sometimes even chronic accompanying pain [Ingraham, 2010]. This is because mitochondria carry their own genetic information and thus do not show the same markers on their surface that would identify them as a body’s own cell. Outside of a body cell, to the immune system, they look like pathogens. But despite being annoying or even torturing when it comes to chronic pain, it is a very safe approach: if something happens and cells are destroyed, from the perspective of our body it looks like something harmful must be going on, such as an injury or infection.
While it is our first and most important defense, the drawback of the innate immune system is that it is non-specific. That means that it has to somehow figure out whether something belongs in the body or not and it might also accidentally target healthy cells. And while this system is relatively fast in its initial response, it still takes some time for each pathogen.
The Adaptive Immune System
What is a more efficient system to distinguish between friend and foe than to look at each case separately?
To answer this, let us look at the basic problem the immune system has to solve. It needs to protect us from pathogens, so the simple answer would be to attack anything that is not the “self.” You could compare it with the police who look for a certain criminal. They have the fingerprints and are selective about who to take to prison and not level the whole neighborhood in the hope that doing so will also hit the criminal. Whether real or the innate, body “police” have to accurately differentiate between friend and foe. The innate immune system does not have such precision, though. While it follows a set of complex rules about how to generally differentiate between its own cells and pathogens, this process takes time—valuable time during which a pathogen could multiply. One solution to speed things up could be to give every cell the same identifier (unique for the person but identical for each cell). This would expedite detection and the immune system could simply try to destroy anything that does not carry such a marker. Using again the example of the police, it is comparable to having the exact address of a criminal versus having to control all people on the street to find the person in question.
Instead of spending time identifying individual pathogens, the adaptive immune system produces pathogen-specific antibodies that bind to specific pathogens.
A Game of Numbers
How can complex organisms beat the evolutionary adaption speed of pathogens?
Unfortunately, the simple approach outlined above would ultimately not solve the problem. It is true that such an immune system offers an identification of “self” and would eradicate most of the pathogens invading our bodies. But a few pathogens might happen to have the same or similar markers as our cells and thus can better hide than others from our immune system. They will multiply and ultimately pose as one of our own cells after a few generations of bacterial or viral infection. And we have to remember that a pathogen evolves very rapidly: for a pathogen, procreation takes hours, while for humans, it takes 20 years from birth to maturity.
The alternative would be to give each body cell a unique identifier to make it very difficult for a virus to spread swiftly throughout your body or pose as a body cell. Unfortunately, this is impossible because it would require storing more than 10,000,000,000,000 (the estimated number of cells in our body) different identifiers in some kind of “police database” of each immune cell. Lacking such a capability, how did nature restore the balance of power in complex life forms with relatively long generation cycles?
Nature had one last trick up its sleeve. It went all in. Instead of trying to compete with pathogens by trying to identify itself, it accepts that pathogens can quickly adapt to one’s cells and targets everything but the self. This also had the advantage of having a “positive” test, meaning that in the worst case a pathogen is not identified as harmful (as opposed to having body cells being destroyed because they are not recognized as innocuous). Now, that sounds like a simple thing to do, but there are around 10,000,000,000,000,000 combinations of proteins that would have to be recognized by the immune system—1,000 times more than we have cells. Likewise, the body has only around 1,000,000 proteins which could be used to recognize those 10 quadrillion proteins or protein combinations of pathogens. In an evolutionary race, we would be hopelessly lost: this number of combinations is impossible to encode into our genetic code (we have only around 3 billion base pairs, or 20,000 genes).
Instead of chaining the immune system cells to our DNA and our long generation cycles, each immune cell is different. It has a variable sequence in its DNA which results in the ability to recognize specific protein structures. Those immune cells that were successful at targeting and eliminating a pathogen were selected, and those immune cells that targeted body cells or did not target pathogens were removed from the immunological gene pool. Over time—during an infection—the immune cells become more efficient through the process of evolutionary selection. During an infection, the memory of successful immune cells is saved for possible later infections in specialized cells, providing a possibly life-long immunity.
With each immune cell being different, the immune system runs an evolutionary selection process against pathogens. This way, our immune system is able to catch up with the rate of evolutionary changes of pathogens.
This was the beginning of the evolution of a mechanism that made it possible for evolution to happen within an organism itself and on smaller timescales, thus outrunning the viral or bacterial evolution. What is remarkable is how a principle like evolution shows up in itself. It could be called a moment of self-reflection: it is the story of the process of evolution discovering that evolution itself is the best way to adapt to an ever-changing environment.
As with everything in nature, there was no sudden appearance of the adaptive immune system. It built upon all the mechanisms that came before. We see few remnants of the previous immune systems in our body. This is simply due to the fact that it is constantly being optimized. Every generation does it a little bit better and you never really see any jump that suddenly introduces a completely new system. The key to understanding evolution is noticing how all the intermediary steps improved the system.

Let us now look at how, specifically, the adaptive immune system works in the body. Please note that this is a simplified explanation. See also Figure 4.25 and 4.26 for an illustration of the process.
- Macrophages traverse through the body, eliminate pathogens they encounter, and present their protein structures (“antigens”) on their surface (innate immune system).
- “T-cells” with specific receptors dock with the macrophage and activate “B-cells” and present the antigen to B-cells.
- The activated B-cells dock with both the T-cells and with the pathogen. Then, B-cells divide into memory cells as protection for later infections as well as antibody-producing plasma cells. In that regard, T-cells act as an additional layer of security to make sure that the B-cells really docked with a pathogen. Also, B-cells do not undergo deselection. Without T-cells, B-cells acting on their own would also produce antibodies against one’s own body cells.
- The plasma cells produce antibodies. Antibodies are not cells but specialized proteins that can dock with specific pathogens and can be produced in large numbers.5.The antibodies dock with the antigens of pathogens and in this way mark the pathogens for destruction. Each antibody can identify a number of similar three-dimensional protein structures.

Clonal Selection
We have already noted that with the adaptive immune system, all your cells carry a unique marker. T-cells originate from stem cells in the bone marrow and are developed in the thymus to have a random configuration. That means that they can recognize different markers with a different efficiency. But before being sent into the bloodstream, and potentially mistaking your body cells as foreign pathogens, all those that actually do dock with body cells (usually around 95%) are sorted out and discarded (“deselected”).
Once out in the bloodstream, a T-cell that docks successfully with a pathogen will multiply. This is the second part of the evolutionary process: antigen-specific T-cells that recognize pathogens faster will increase in number, getting faster and faster with each generation, until the adaptive immune system is so fast that it is called “immune” against a pathogen. So, we can still be infected by diseases to which we have immunity, but the adaptive immune system then works so quickly at preventing any significant spread that we do not notice.
Applications
Autoimmune Diseases: If something goes wrong during this deselection process, autoimmune diseases like diabetes, multiple sclerosis, rheumatoid arthritis, etc. can occur. Likewise, if children do not encounter certain pathogens early in life, allergies can be the result where the immune system thinks that harmless plant or animal proteins are actually dangerous pathogens that deserve a full immune response. Obviously, our immune system also makes it difficult for whole foreign organs with different markers to be transplanted into the body: without additional medication to suppress the adaptive immune system, transplanted organs are rejected. Knowing how our immune system works and understanding how it originally developed will help us in the future to treat autoimmune diseases.
Vaccination: If the same or a similar pathogen invades the system a second time, the body can reuse existing memory cells which can then immediately start producing antibodies specific to the pathogen. This speeds up the immune response because it saves the time of having to adapt to the specific protein structure again. This opens the door for vaccination: injecting deactivated pathogens lets the immune system create memory cells while not being in danger of a pathogen actually infecting the body. As opposed to this, so-called “active immunization,” alternatively, involves specific antibodies being injected. With “passive immunization,” the body develops an immunity, but it is only temporary because no memory cells are created and the antibodies will sooner or later vanish.
Computer Security: For protecting computers or networks against malicious programs, a similar, albeit less complex, system is used. The system is tested at its normal “clean” state of operation and the state of the parameters are memorized by the machine. The “immune cells” of a computer security program would have to be flexible to recognize different patterns of use. For example, a website might get significantly more visitors during the day than during the night, but that should not be evaluated as a break-in attempt or “denial of service” attack. Likewise, it needs to be specific to recognize known pathways of attack, like accessing certain network addresses or trying to change sensitive parts of the operating system.
Summary:
Instead of spending time identifying individual pathogens, the adaptive immune system produces pathogen-specific antibodies that bind to specific pathogens.
With each immune cell being different, the immune system runs an evolutionary selection process against pathogens. This way, our immune system is able to catch up with the rate of evolutionary changes of pathogens.
With the immune system, we have seen the power of the idea of evolution: it is so ingenious that lifeforms evolved to have within themselves mechanisms that resemble evolution. And not only can we learn more about evolution from the study of our immune system, but we can also draw analogies and parallels to security systems of any kind, be it online or in the real world. We could draw the conclusion that a system of police that takes great care of the innocent and precisely targets criminal is most effective. On the other hand, even such an advanced system as the adaptive immune system can run amok and target itself (autoimmune disease), just like an effective police force can end up being used against its own country. Our bodies have evolved to strike a balance between an innate immune system that might be too indiscriminate and an adaptive system that might turn against itself.